“Train, Baby, Train” and the Nuclear SMR Challenge
In the second part of our piece dedicated to the challenges ahead for the nuclear industry in 2025, let's try to understand why was the West delayed regarding SMRs by looking back at the 1960s.
OPINIONNUCLEAR
Atom News
2/18/20255 min read
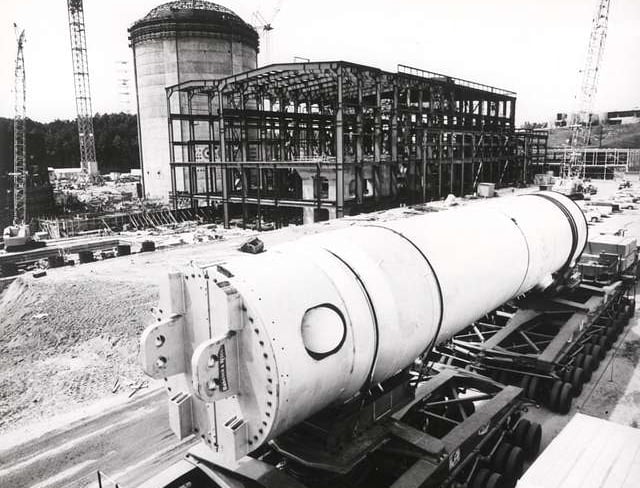
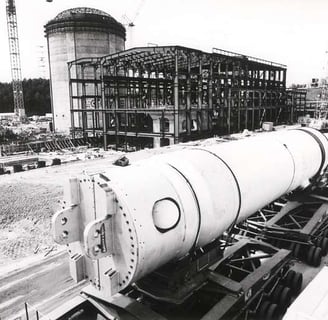
Hello everyone! And welcome back on Atom News for the second article of a three-part piece we dedicate to the nuclear challenges of 2025. And beyond.
Today on the menu: humble pie. We drive you through the quest for a closed fuel loop and SMRs and into the why of the West’s delay.
First let’s dive back in time to the heydays of nuclear energy. Picture that: we’re in the sixties. Civilian use of nuclear power is booming. Crazy scientists work feverishly on various projects to better harness the power of atom. The consensus quickly coalesced around the design that everybody uses today. A thermal neutron reactor, using low-enriched uranium and steam to power its turbines.
But there were others.
These designs were not as conventional as the one we know today. Here’s some quick tablecloth physics for you. At its natural state, uranium is made up of U238, which is not fissile, and a minute trace of U235, which is. To be able to sustain fission, fuel is enriched at around 5% U235, which is enough to last a good 12-to-18 months in a reactor. To initiate fission, a fissile element like U235 needs a neutron. But to be able to properly split it, said neutron need to be slowed – “moderated”, otherwise it misses the mark. We call these neutrons “thermal”. This name is rather misleading, because it also generates less heat. You don’t need enormous heat to boil water after all, and too much of it could make it difficult to keep your primary cooling loop in a liquid state.
Problem is, when your U235 gets all split up in the reactor core, you get on the one hand a mix of “depleted” uranium and plutonium, and on the other hand nasty transuranic (read heavier than uranium) elements like curium and americium. The former's need to be separated and re-enriched to have another shot at fission. The latter… Well, they get buried deep underground with the hope that no one will be curious enough to try and dig it up for the rest of their long, long half-life.
But what if you used something else to cool your reactor, something that doesn’t boil at 100 degrees?
Then you could turn up the heat, and opt for not slowing your little neutron friends. And that’s when it becomes interesting. See, unmoderated, “fast” neutrons have a nice property. They can interact with other elements than plain ol’ U235. Being fast and punchy, they can go play with U238 and Thorium. These elements have a nice property too: that of being able to gobble up a neutron, to turn into… a fissile isotope.
Yup, fast neutrons can in fact generate “fresh” fuel and burn down the nasty and heavy radioactive transuranic elements that we mentioned earlier. A tandem of thermal and rapid neutron reactors could theoretically make a set supply of uranium last – if not forever, at least a lofty number of centuries.
However, these designs require some rather unconventional coolants: liquid sodium, molten lead, gas. Elements that do not turn to gas (or already are) at high temperature and that do not slow neutrons down when they pass through it. This meant that research for thermal and rapid reactors were two different fields. Highly complementary, but requiring different sets of expertise and heavy investment to properly develop.
And that spelled doom for our rapid friends.
The incidents of Chernobyl and Three Miles Island sent the initial fervor for nuclear energy in a downward trend. Coupled with various recessions and a rather ample supply of uranium (which didn't warrant the development of reactors that could reuse spent fuel), the collective West, from the US to France, slowly let go of their R&D effort on rapid reactors to focus on more proven designs: full-scaled thermal reactors, known today as the “third” generation of reactors.
But some did persevere. In Russia and China, programs were maintained. Budgets and engineers were kept. With tangible results. Fast forward to today, as the issue of nuclear waste is starting to become real and uranium is becoming a strategic resource (go read our first piece for more), having a fully-closed fuel cycle could mean complete autonomy from the turpitude of the world uranium market, as well as cutting by a factor of a hundred the amount of waste you ultimately have to bury. That’s what we call… the “fourth gen”. Whereas the third generation sought to maximize reactor safety, the fourth aims at maximizing fuel efficiency and waste reduction.
And those remotely close to succeed at that are China and Russia.
Russia’s Proryv program, based on VVER thermal reactors and fast, sodium-cooled BN family and lead-cooled BREST reactors is well underway. The BN family boasts functioning prototypes of the 600MW and 800MW sort, with a full-scale 1200MW model under construction in Beloyarsk. BREST is being built in Seversk. Said program is conducted conjointly with China, which in turn sports its CFR-600 reactors on the island of Xiapu, as well as an -almost- functioning HTR-PM reactor in Shidao Bay (a helium-cooled design they borrowed from South Africa, but that’s a story for another time). Opposite to its coastal colleagues, the TMSR-LF1, with a molten salt coolant and thorium-based fuel, is ready and functioning in the remote Gansu province.
This continuous investment in nuclear energy pays double. Indeed, these two countries are on the brink of success whereas most of us Westerners have simply given up. Having an army of nuclear engineers that did not stop working on perfecting their designs means there was virtually no loss of know-how in the course of their nuclear programs. But it also means they have the capacity to be quick to the punch when a new opportunity presents itself.
Small modular reactors are a good example of that. Originally designed for military applications (in subs or aircraft carriers), the idea of creating a miniature nuclear reactor – which goes against the tried-and-true economy of scale principle – was made mainstream by an American university back in the 2000’s. Regulation and proliferation issues notwithstanding, the main issue with these little buggers is they require, even more than their full-scale counterpart, a huge serial effect to be financially viable. Their huge R&D cost means you need to build several to break even. Meaning the first country to offer SMR has a good chance to bury its competitors.
Can you make a guess who has the most advanced design today?
Yup. Thanks to their robust research program, China’s ACP100, and Russia’s RITM200, respectively seated on the Hainan Island and Ust-Kuyga, are on their way to become world first. The myriad of Western start-ups vying to offer a credible design, not to mention a working prototype, would be hard pressed to survive if these two hit the market. Thankfully, nuclear reactor markets tend to be quite sensitive to geopolitical issues, meaning Europe and the US should still be open for late comers. But chances are there isn’t going to be many spots left for us in less politically charged areas.
Stay tuned for the last part! ⚛️